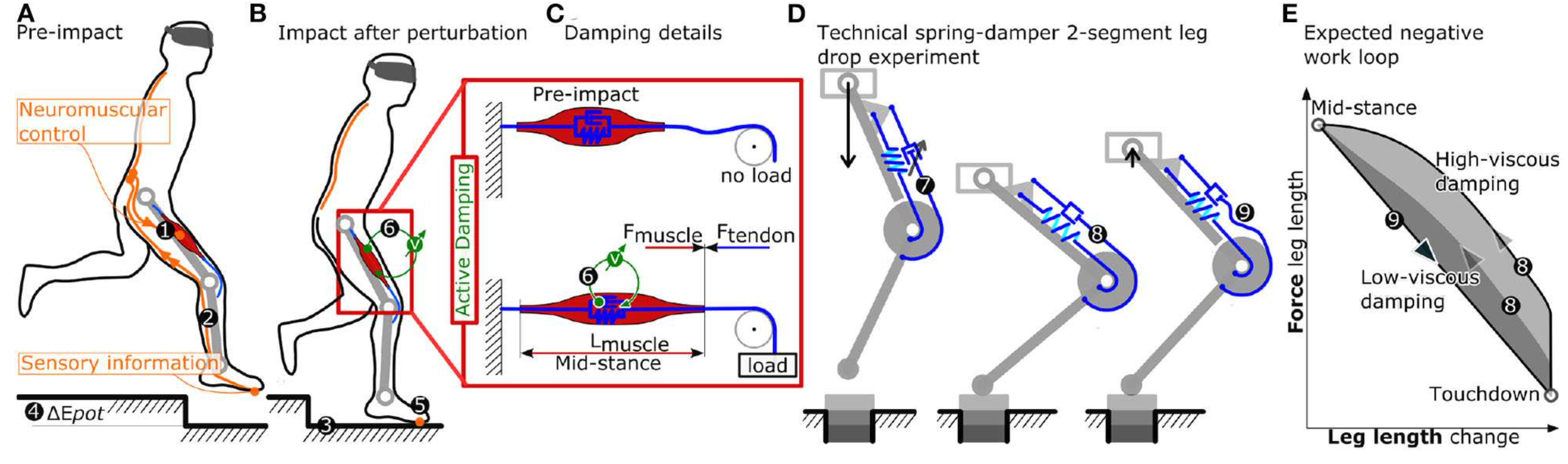
A) The nerve conduction velocity in organic tissue is limited (More et al., 2010). B) In case of perturbations like an unexpected step-down, delayed sensory information can be lead to falling. C) We hypothesize that 'smart' actuators like muscles possess internal mechanisms leading to muscle force based on the muscle extension velocity. D) We implement a two-segmented leg with a parallel spring-damper. A) The nerve conduction velocity in organic tissue is limited (More et al., 2010). B) In case of perturbations like unexpected step-down, delayed sensory information can be lead to falling. C) We hypothesize that muscles possess internal mechanisms creating muscle force based on the extension velocity. D) We develop a two-segmented leg with a parallel spring-damper. E) Energy dissipated by viscous damping.
Muscle models and experimental observations suggest that locomotion stability might benefit from physical, viscous damping in muscle-tendon tissue. Contrary to active damping, physical damping is inert to delays in neuromuscular control, and noise caused by sensing, actuation, or information transmission.
We investigate how to exploit physical damping in a leg drop experiment; the stance phase of legged locomotion can be separated into a drop component and a leg angle component. The numerical simulations suggest that adjustable and viscous damping deals best with ground perturbations, compared to Coulomb damping.
In two hardware implementations we test a commercial, off-the-shelf hydraulic damper, and a custom-made, rolling diaphragm damper. We separate the dissipated energy into its components with the help of a dedicated experimental design. We quantitatively identify energy dissipation from the early impact (unsprung-mass effects), viscous damping, Coulomb damping, and orifice adjustments. Viscous, hydraulic dampers react velocity-dependent, and create an instantaneous, physically adaptive response to ground-level perturbations without sensory input [ ].
In [ ], we present a new simulation model with a damping term that can be adjusted without retuning other model parameters. We compare how increased damping affects stability for unexpected ground-height perturbations. Our measure of task-level stability allows trajectories to be compared quantitatively instead of only being separated into a binary classification of “stable” or “unstable”.
Our simulation study results show that increased damping contributes significantly to task-level stability; however, this benefit quickly plateaus after only a small amount of damping. Low intrinsic damping values observed in experiments may have stabilizing benefits, and are not simply minimized for energetic reasons.