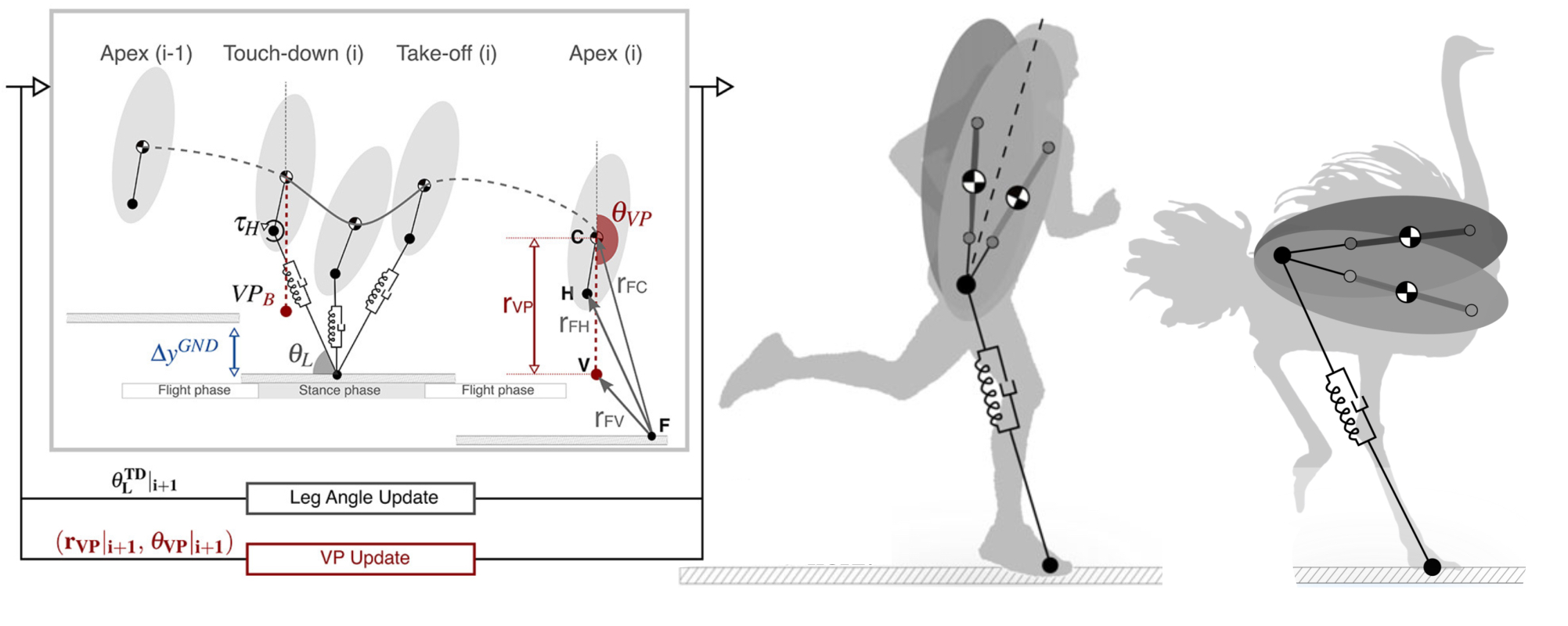
We investigate the functional demands of bipedal running with a focus on trunk orientation and virtual point mechanics. Bipedalism evolved in reptiles, avians, theropods, and primates. Amongst bipeds, birds and humans demonstrate exceptional agility, locomotion efficiency, and terrain traversability despite having limitations in actuation and sensory delays [Alexander, 1992, More et al. 2010]. The trunk accounts for 1/2 the humans’ body mass, and 3/4 of a bird’s mass. With a small support base (feet), the trunk and its orientation become an integral part of bipedal legged locomotion generation, beyond the task of trunk stabilization. We utilize a spring-loaded inverted pendulum model which we extend by a rigid trunk, similar to the T-SLIP model by Maus et al., 2008.
We implement VP controllers with a VP above (VPA) or below (VPB) the center of mass. We research bipedal VP running in the following scenarios: downhill and step-down running for human-like morphology (numerical simulations), human step-down running (experiments and numerical simulations), human running on level ground (numerical simulations), and running with bird-like trunk orientation (numerical simulations) [ ].
We show that positioning the VP below the center of mass (CoM) can explain the forward trunk pitching during human running. We show that the VP method can stabilize single step-down perturbations up to 40cm, and downhill grades up to 20–40 degrees at speeds of 2–5 m/s. A VP below the CoM leads to a synergistic work between the hip and leg, reduces leg loading, but increases peak hip torque. Camouflaged step-down perturbations affect the location of the VPB in simulation and human experiments. Our simulation results suggest that a VPB can encounter the step-down perturbations and bring the system back to its initial equilibrium state. Adjusting the VP vertically redistributes joint loads, and potentially improves locomotion energy efficiency.